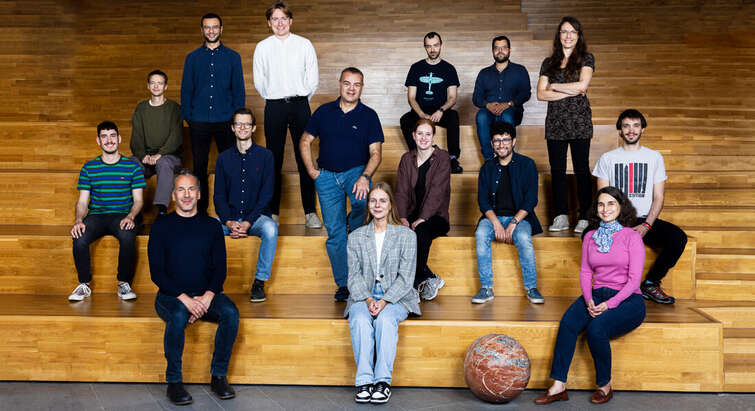
Structural molecular biology in the Montoya Group
The Montoya Group works in the interface between biology, physics and chemistry and uses molecular biology, X-ray crystallography and cryo-electron microscopy to dissect the working mechanisms of the macromolecules that constitute the cell’s machinery.
The Montoya lab of Structural Molecular Biology has established itself in the last 20 years as one of the leading research groups in the field of macromolecular machines involved in cell division, genome maintenance, and their connection with molecular scissors initiating genome editing. My expertise is documented by 122 manuscripts, many in top-level peer-reviewed journals, 50 of them published in the last 10 years. My lab combines X-ray crystallography and cryoEM with biochemical, biophysical, and cellular approaches to gain insight into the molecular mechanisms of these complexes.
We have consistently produced high impact discoveries, such as the first crystal structure of the 1 MDa TRiC/CCT chaperonin in complex with tubulin (Muñoz et al Nature Structural & Molecular Biology 2011). This structure sheds light on CCT substrate recognition and folding mechanism, unveiling possible therapeutic vulnerabilities in cancer.
My group is also systematically pursuing structure–function analysis of endonucleases, which are of great interest because of their applications in biomedicine and biotechnology. We showed that redesigned homing endonucleases correct mutations causing human monogenic diseases, thus opening new therapeutic avenues (Redondo et al, Nature 2008; Molina et al Nature Structural & Molecular Biology 2015, Marcaida et al, PNAS 2008; Lopez et al PNAS 2007, Muñoz et al, NAR 2012). We have also elucidated the mechanism and further redesign of Cas12a, a new CRISPR-Cas effector ribonucleoprotein complex, unveiling the mechanism of recognition, unzipping, and cleavage of the target DNA (Stella et al, Nature 2017, Stella et al., Cell 2018, Paul NAR 2021).
Our studies have generated variants of Cas12a for therapeutic applications, which are being developed by Twelve Bio, a spin-off company from my lab (see CV). In addition, we have recently determined the structure of the largest CRISPR-Cas effector complex to date, Cmr-beta (ca. 1 MDa), which contains 6 different catalytic centres showing mechanistic analogies of Type III CRISPR-Cas to the innate immune system. We contribute reviews and opinions to leading journals (Nature Structural & Molecular Biology 2017, Current Op. Struct. Biol. 2020). These achievements were recognized by prizes to G. Montoya (see CV) as well as by multiple fellowships from top agencies to students and postdocs in the lab (MSCA, HFSP, EMBO, Lundbeck).
- Structure of the TnsB transposase-DNA complex of type V-K CRISPR-associated transposon. Tenjo-Castaño F, Sofos N, Lopez-Mendez B, Stutzke L, Fuglsang A, Stella S, Montoya G. (2022) Nature Comms Oct 2;13(1):5792. doi: 10.1038/s41467-022-33504-5.
- Structure of the RAF1-HSP90-CDC37 complex reveals the basis of RAF1 regulation. García-Alonso S, Mesa P, Ovejero LP, Aizpurua G, Lechuga CG, Zarzuela E, Santiveri CM, Sanclemente M, Muñoz J, Musteanu M, Campos-Olivas R, Martínez-Torrecuadrada J, Barbacid M, Montoya G. (2022) Molecular Cell 5;82(18):3438-3452.e8. doi: 10.1016/j.molcel.2022.08.012
- Structure of the mini-RNA-guided endonuclease CRISPR-Cas12j3. A. Carabias del Rey, A. Fulgsgang, P. Temperini, T. Pape, N. Sofos, S. Stella, S. Erlendsson, G. Montoya (2021). Nature Comms 22;12(1):4476. doi: 10.1038/s41467-021-24707-3.
- Structures of the Cmr-β Complex Reveal the Regulation of the Immunity Mechanism of Type III-B CRISPR-Cas. Sofos N, Feng M, Stella S, Pape T, She Q and Montoya G (2020) Molecular Cell 3;79(5):741-757.e7. doi: 10.1016/j.molcel.2020.07.008
- Conformational Activation Promotes CRISPR-Cas12a Catalysis and Resetting of the Endonuclease Activity. Stella S, Mesa P, Thomsen J, Paul B, Alcón P, Jensen SB, Saligram B, Moses ME, Hatzakis NS, Montoya G. (2018) Cell 13;175(7):1856-1871.e21. doi: 10.1016/j.cell.2018.10.045
- Molecular basis of Tousled-Like Kinase 2 activation. Mortuza GB, Hermida D, Pedersen AK, Segura-Bayona S, López-Méndez B, Redondo P, Rüther P, Pozdnyakova I, Garrote AM, Muñoz IG, Villamor-Payà M, Jauset C, Olsen JV, Stracker TH, Montoya G. (2018) Nature Comms 28;9 (1):2535. doi: 10.1038/s41467-018-04941-y.
- Class 2 CRISPR-Cas RNA-guided endonucleases: Swiss Army knives of genome editing. Stella S, Alcón P, Montoya G. (2017) Nature Structural & Molecular Biology 24 (11):882-892. doi:10.1038/nsmb.3486.
- Structure of the Cpf1 endonuclease R-loop complex after target DNA cleavage. Stella S, Alcón P, Montoya G. (2017) Nature 22; 546(7659):559-563. doi: 10.1038/nature22398
- Crystal structure of the mammalian cytosolic chaperonin CCT in complex with tubulin. Muñoz, I.G., Yébenes, H., Zhou M., Mesa, P. Serna, M. Bragado-Nilsson, Beloso, A., E. de Carcer G., Malumbres M., Robinson C.V., Valuesta, J.M. & Montoya G. (2011) Nature Structural & Molecular Biology Jan;18(1):14-9. doi: 10.1038/nsmb.1971.
- Molecular basis of recognition and cleavage of the human Xeroderma pigmentosum group C gene by engineered homing endonuclease heterodimers. P. Redondo, J. Prieto, I. Muñoz, A. Alibés, F. Strichter, L. Serrano, S., P. Duchateau, F. Paques, F. Blanco & G. Montoya. (2008) Nature 6;456(7218):107-11. doi: 10.1038/nature07343.
Staff list
Name | Title | Phone | |
---|---|---|---|
Arturo Carabias del Rey | Researcher | +4535337930 | |
Elisabeth Bragado Nilsson | Research Technician. | +4535330659 | |
Francisco Javier Tenjo Castano | Academic Research Staff | +4535336501 | |
Guillermo Montoya | Professor, Head of Research | +4535330663 | |
Magdalena Masternak | Postdoc | +4535332143 | |
Nicholas Heelund Sofos | Microscopy specialist | +4535328272 | |
Pablo Mesa | Research Consultant | +4535333636 | |
Ricardo García Martín | PhD Fellow | +4535329629 | |
Sanjay Dey | Postdoc | +4535322013 | |
Tillmann Hanns Pape | Microscopy specialist | +4535326593 |